Erich Mueller
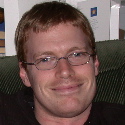
- B.Sc., Mathematics/Physics, 1996, University of British Columbia.
- Ph.D., Physics, 2001, University of Illinois at Urbana Champaign.
- Postdoctoral fellow, Physics, The Ohio State University 2001-2003.
- Assistant Professor, Physics, Cornell, 2003-2009.
- Associate Professor, Physics, Cornell, 2009-2015,
-
Professor, Physics, Cornell, 2015-present.
- Alfred P Sloan Fellow 2005-2007
- Robert A and Donna B Paul Award for Excellence in Advising 2013,
- APS Fellow 2014.
- Cornell Physics, Director of Undergraduate Studies 2009-2013.
- Laboratory of Atomic and Solid State Physics 2015-present.
Research Statement
Like many academics, I am attracted to puzzles. I like coming face-to-face with apparent paradoxes, and explaining counterintuitive phenomena. Reflecting these interests, I have made a career out of studying quantum mechanics: the physics on the scale of single atoms, where classical mechanics fails. My research is focused on understanding how the theory of quantum mechanics manifest itself. I am particularly interested in the cases where simple interactions between a collection of particles leads to complicated behavior. Much of our technology is based on such emergent phenomena (electronics, lasers...), and I am always alert to possible applications of my work to areas such as metrology. My motivation, however, is closer to that of an explorer than that of an inventor. I want to see new things.
Much of my efforts are directed at understanding the behaviors of alkali gases cooled to nano-Kelvin temperatures. One cools these gases in order to eliminate the random thermal motion which dominates at room-temperature. One is then able to see the underlying quantum behavior of the atoms. As a simple example, consider one of the central tenents of quantum mechanics: the Heisenberg uncertainty principle. This principle states that one cannot simultaneously know where an object is and how fast it is moving (or more precisely that the product of the uncertaintly in position and momentum is bounded below by a fundamental constant). As one cools a gas, one is reducing the uncertainty in the speed of the particles, and hence their positions become less certain. In other words, quantum mechanics predicts that at low temperatures atoms become fuzzy, and that the colder they are the fuzzier and more spread-out they become. When the atoms are so cold that they begin to overlap, a qualitative change occurs to the gas: one no longer can tell where one atom ends and the next one begins. Effectively each atom becomes delocalized over the entire cloud, forming what is known as a condensate. For a typical cold atom experiment, this means atoms are in quantum superpositions of being in locations whose separations exceed 1 mm. I have been studying the consequences of such non-classical phenomena.
Some of my research is driven by asking fundamental questions. For example, the scenario described above applies to a gas of featureless atoms with no internal degrees of freedom. In sufficiently low fields most atoms have nuclear and electronic spins which can point in any direction. For such spinor gases, should one find a single condensate upon cooling? As was pointed out in the early 1980's, it is in principle possible to simultaneously have several condensates, each with the spins pointing in different directions.
The answer to how many condensates are formed
depends on an interplay of the atomic interactions and magnetic field gradients.
With my collaborators, I have investigated this issue, relating the
I also carry out more experimentally driven research. For example, I have had close collaborations with Randy Hulet's group at Rice, and Brian Demarco's group at UIUC.
One of my current long-term goals is finding paradigms for understanding non-equilibrium physics in isolated quantum systems -- This has both practical and fundamental importance. On the practical side, the biggest obstacle to progress in cold atoms comes from various sources of heating, and difficulty in cooling strongly interacting gasses. Only through a thorough understanding of the kinetics of these quantum systems can we hope to overcome these obstacles. On the fundamental side, there have been a number of debates about the conditions for equilibration of isolated quantum systems, and how the system reaches steady state. Do the quantum correlations of the initial state persist? Not only is this interesting for engineered systems, but it may have relevance for the dynamics of the early universe. My group is addressing this issue by modeling various non-equilibrium experiments (each of which is interesting in their own right). We have made progress on several of the practical issues (for example making predictions that certain atomic species are more favorable than others for experiments with light-induced spin-orbit coupling). In the near-term, I want to address this problem by playing with various quantum Boltzmann equations. Our group has been corresponding with experimentalists at MIT, Chicago, and UIUC who are doing experiments which can validate these equations. My philosophy here is to see how far one can push the simplest methods -- gradually building sophistication as needed. To contrast this, there are a number of theorists who take the philosophy that you start with an exact formulation (typically a black-box numerical technique like DMRG), and then make hypotheses based on numerical experiments. Both can be effective approaches to science.
A second long-term goal is to find novel quantum states of matter. This has been an ongoing goal of mine, directly resonating with my "explorer" mentality. I expect this to be a never-ending quest, with projects inspired by new experimental capabilities and new theoretical inspirations. For example, recently we have done a fair amount of work inspired by "topological insulators." In the near future, I have ideas related to fractional quantum Hall effects, cold atom analogs of heavy fermion superconductors, and "modulated superconductivity" (FFLO). I truly believe that all of these will be seen with cold atoms, and that in the search we will learn fundamental truths about the quantum mechanics of interacting particles.
Teaching Statement
I have a strong commitment to teaching, both at the graduate and undergraduate level. I have been involved in teaching a number of graduate and undergraduate courses at Cornell, and have helped with several major educational initiatives. I see teaching as a team effort, involving everyone in the Cornell community. I believe learning should be student-centered, and strive to empower my students. One should not be content with the status quo, but should always be improving the teaching environment. One of the joys of being at Cornell is that there is a strong community which is enthusiastic about improving the education of students. I appreciate being part of this community and having the opportunity to excel in all of my professional activities.